Genetic testing to modulate when to operate in thoracic aortic disease
Introduction
Thoracic aortic aneurysm (TAA) can be fatal if it progresses asymptomatically until a later stage when dissection and rupture occur. Treatment methods for thoracic aortic aneurysm and dissection (TAAD) include both medical therapy and surgical repair. Medical therapy to prevent growth, rupture, and dissection of TAAs—including β-blockers and angiotensin receptor blockers (ARBs)—have been largely disappointing (1). Surgical repair is generally performed when the aortic diameter reaches 5.5 cm (2) but adjusted recommendations have been proposed based on clinical and molecular genetics. This monograph reviews the appropriate modulation of surgical criteria based on clinical and molecular genetics.
Categorization of TAAD
Family history, specifically the presence of an affected first-degree relative, is a crucial factor in the family history of the TAAs, as it suggests a strong genetic component in TAAD development. These studies show that a remarkably consistent 21% of patients with TAA have a family member with a known aneurysm somewhere in the body (3).
Familial TAA can be divided into two such categories: syndromic and non-syndromic. Syndromic aortic aneurysm connotes involvement of multiple organ systems and normally presents at an earlier age due to the severity of the phenotype. Patients with syndromic aneurysms are easier to identify based on the overt manifestations of genetic diseases with generalized defects in connective tissue. Syndromic TAA disease includes Marfan syndrome (MFS), Loyez-Dietz syndrome (LDS), Ehlers-Danlos syndrome (EDS), and arterial tortuosity syndrome (ATS).
Non-syndromic TAAD is characterized by involvement of aortic tissue only. This can be divided into two subcategories: familial, which refers to more than one member of the family affected, and sporadic—without affected family members. Familial disease is usually clinically silent and difficult to detect until a later stage. Therefore, the ability to utilize genetic testing for detection, characterization and treatment recommendations of TAAD represents a major advance in patient care. We expect an increase in genetic testing and continued novel gene polymorphism identification as genetic understanding burgeons.
Syndromic TAAD
MFS
Hundreds of specific mutations in the FBN1 gene have been found to cause MFS. Due to the virulence of this disease (potential for catastrophic aortic dissection), the current recommendation is to operate when the aneurysm reaches >5.0 or >4.5 cm for patients with familial history of aortic dissections. Rapid growth (more than 0.5 cm per year) as well as the presence of significant aortic valve regurgitation also encourage early operation (2). Specific recommendations for female MFS patients planning on pregnancy stipulate surgical repair of the aortic root and ascending aorta at the size of 4.0 cm (4). Moreover, if the patient is undergoing aortic valve replacement for aortic insufficiency, it is recommended to repair ascending aortic aneurysm if the diameter exceeds 4.5 cm (2). Similar guidelines are recommended for pediatric MFS patients, who should undergo aortic aneurysm repair if the aortic diameter is greater than 5.0 cm, if there is a rapid growth of 0.5 cm per year, or if aortic regurgitation is present (5).
LDS
LDS is another genetic connective tissue disease within the syndromic TAAD category. It is inherited in an autosomal dominant manner and can be distinguished from MFS by the presence of unique features such as bifid uvula, cleft palate and hypertelorism (6,7). This disease is primarily associated with heterozygous mutations in the transforming growth factor-β receptor 1 and 2 genes (TGFBR1 and TGFBR2, respectively) and is characterized by a more severe vascular phenotype with dissections and ruptures happening at smaller aortic diameters (7). Although these two receptors contribute to the same TGF-β signaling pathway, they tend to affect the patient population in different ways. For instance, female patients with TGFBR1 mutation tend to have lower risk of the aortic dissection than male patients with the same mutation, whereas those with TGFBR2 mutation do not demonstrate the same sex preference (8). In addition to the risk difference, the aortic size at dissection also tends to be unequally distributed between the two sexes. Female patients with the TFGBR2 mutations, but not TGFBR1, have had dissections with an aortic diameter of less than 4.5 cm. Men, on the other hand, did not generally experience dissections below 4.5 cm with TGFBR1 or TGFBR2 mutations (7) (Table 1).
Table 1
Sex | Mutation | |
---|---|---|
|
|
|
Male | Higher risk of aortic dissection | <4.5 cm aortic size at dissection |
Female | Lower risk of aortic dissection | >4.5 cm aortic size at dissection |
LDS, Loyez-Dietz syndrome.
Based on these observations, patients with these mutations should consider surgery when the aortic diameter is 4.5 cm, also women with the mutations in the TGFBR2 gene should consider surgical repair when aortic size is 4.0 cm, especially if severe phenotypic features are present such as aortic tortuosity and hypertelorism. On the contrary, women with TGFBR1 mutations can be subjected to less stringent criteria for surgery in the absence of familial history of aneurysm or drastic increase in the diameter of the aorta (7).
As more genes have been identified, the molecular profile of LDS has been expanded. Currently, this disease is subcategorized into six subtypes depending on the specific gene mutations identified. LDS type 1 patients are denoted by TGFBR1 mutation, LDS type 2 presents with the TGFBR2 mutations, LDS type 3 has mutations in the SMAD3 gene, type 4—TGFB2, type 5—TGFB3 and type 6—SMAD2 (7) (Table 2). All of these genes contribute to the same TGF-β signaling pathway.
Table 2
Type | Gene | % of cases | Diameter at which to operate (cm) |
---|---|---|---|
Type 1 |
|
20–25 | 4.0–4.5 |
Type 2 |
|
55–60 | 4.0–4.5 |
Type 3 |
|
5–10 | 4.0–4.2 |
Type 4 |
|
5–10 | 4.5–5.0 |
Type 5 |
|
1–5 | 5.5 |
Type 6 |
|
1–5 | 5.5 |
LDS, Loyez-Dietz syndrome.
TGF-β is a cytokine recognized by the TGFBR receptor and SMAD is a downstream effector molecule. Despite working through the common signaling pathway, these mutations have different prevalence rates among patient population. The majority of patients with LDS present with mutations in TGFBR1 (subtype 1, 20–25% of cases) and TGFBR2 (subtype 2, 55–60% of cases). This may be explained by a more drastic effect that the mutation of the upstream receptor may have on the overall signaling pathway.
The general surgical treatment recommendation for LDS patients is indicated surgery when the internal aortic diameter is >4.2–4.5 cm determined by the transesophageal echocardiogram or if external diameter is 4.4–4.6 cm shown by the computed tomographic imaging and/or MRI (2). As more knowledge has developed regarding molecular biology of the potential gene mutations involved in each individual subtype, more specific guidelines have been articulated. For instance, patients with LDS types 1, 2, 3 are known to have dissection when the diameter reaches 3.9–4.0 cm (6), whereas patients with LDS type 4 have dissection when the diameter is between 4 and 5 cm (9). Therefore, it is recommended to perform surgery for patients with LDS types 1 and 2 when the diameter is 4 cm (10). Patients with LDS type 3 and mutations in SMAD3 gene should undergo surgical repair when the ascending aortic size is 4.0–4.2 cm (11). Patients with LDS type 4 are recommended surgery when the diameter is 4.5–5.0 cm (10), whereas patients with LDS types 5 and 6 should follow standard recommendations for surgical repair (Table 2).
Additionally, specific recommendations have been made for various LDS types in different age categories. For pediatric patients, the threshold of 4.0 cm should be used for children with a slowly growing aneurysm. Children with severe craniofacial malformations should undergo surgery when the aortic root z-score is >3.0 or if a growth rate greater than 0.5 cm per year is detected (11). However, surgery can be delayed until the size of the annulus is 1.8 cm, which would allow for a simultaneous placement of a valved graft that will accommodate rapid growth. Pediatric patients with mild craniofacial malformations should undergo surgery when the aortic root z-score is greater than 4 or if a growth rate greater than 0.5 cm per year is detected, or if there is significant aortic regurgitation (10,11). For adult patients with LDS types 1–3, surgery is recommended at >4.0 cm in ascending aortic aneurysm and aortic root aneurysm as well as if the aorta is rapidly expanding (0.5 cm over 1 year) (10). Moreover, since adult patients with the LDS type 4 develop dissection with the aortic diameter as low as 4.0 cm (10), monitoring should take place within the 4 cm range and patients should receive surgical repair at the size of 4.5–5.0 cm (12).
EDS
The vascular subtype of EDS is characterized by mutations in COL3A1, the gene that encodes collagen, a major component of the blood vessel wall. Since clinical presentation of patients with EDS is very similar to that of MFS and LDS, the precise diagnosis is usually confirmed by sequencing the COL3A1 gene. Surgical repair recommendations in this case are stringent due the fragile nature of the aortic tissue which can, in some cases, be unworkable (2). However, surgery should be performed in the event of arterial rupture, unstable aneurysms, dissecting aneurysms that affect the aorta or iliac vessels, or when the aortic diameter reaches 4.5–5 cm range (13). Vessel friability is the key factor to be considered intraoperatively in order to decrease morbidity in EDS patients.
ATS
ATS is an autosomal recessive connective tissue disease characterized by mutations in the gene encoding glucose transporter SLC2A10 (14). Very few aortic mutations are recessive. The impaired transport of glucose, which is the main source of metabolic energy in the human body, leads to weakness of the blood vessel wall and causes aneurysmal dilation and focal stenosis (15). Additionally, recent studies in mice have demonstrated that mutations in this gene are associated with an increased production of the reactive oxygen species (ROS) that are known to cause oxidative damage to the aortic tissue (16). The ATS mortality rate is relatively high for younger patients: 40% for children under 5 years old. The severity of this condition is due to vascular insufficiency that is caused by fragmentation of elastic membrane and fibers (17). A recent study examining phenotypic effects of SLC2A10 in three families implies that TAAD may not be a key feature of ATS as only 3 patients had developed aortic aneurysm and no dissections were reported (18). Despite rare occurrence of TAAD in ATS, surgery recommendations remain standard and repair should be performed when the aortic diameter reaches 5.5 cm (13).
Non-syndromic TAAD
As of now, 30 genes have been identified to contribute to TAAD development, mainly through their impact on the extracellular matrix modifications, TGF-β signaling pathway dysregulation and changes in smooth muscle contraction (Table 3) (12). Specific mutations contribute to the severity of TAAD and confer distinct recommendations regarding surgical intervention (Figure 1) (12).
Table 3
Gene | Protein | Animal model leading to vascular phenotype? | Syndromic TAAD | Non-syndromic FTAAD | Associated disease/syndrome | Associated clinical characteristics of the vasculature | Ascending aorta size (cm) for surgical intervention | Mode of inheritance | OMIM |
---|---|---|---|---|---|---|---|---|---|
|
Smooth muscle α-actin | Yes | + | + | AAT6 + multisystemic smooth muscle dysfunction + MYMY5 | TAAD, early aortic dissection, CAD, stroke (moyamoya disease), PDA, pulmonary artery dilation, BAV | 4.5–5.0 | AD | 611788, 613834, 614042 |
|
Biglycan | Yes | + | − | Meester-Loeys syndrome | ARD, TAAD, pulmonary artery aneurysm, IA, arterial tortuosity | Standard | X-linked | 300989 |
|
Collagen 1 α2 chain | No | + | − | EDS, arthrochalasia type (VIIb) + cardiac valvular type | Borderline aortic root enlargement | Standard | AD + AR | 130060, 225320 |
|
Collagen 3 α1 chain | Yes | + | − | EDS, vascular type (IV) | AAD, early aortic dissection, visceral arterial dissection, vessel fragility, IA | 5.0 | AD | 130050 |
|
Collagen 5 α1 chain | No | + | − | EDS, classic type (I) | ARD, rupture/dissection of medium sized arteries | Standard | AD | 130000 |
|
Collagen 5 α2 chain | Partially | + | − | EDS, classic type (II) | ARD | Standard | AD | 130000 |
|
Fibulin-4 | Yes | + | − | Cutis laxa, AR type Ib | Ascending aortic aneurysms, other arterial aneurysms, arterial tortuosity and stenosis | Standard | AR | 614437 |
|
Elastin | No | + | − | Cutis laxa, AD | ARD, ascending aortic aneurysm and dissection, BAV, IA possibly associated with SVAS | Standard | AD | 123700, 185500 |
|
Elastin microfibril interface 1 | No | + | − | Unidentified CTD | Ascending and descending aortic aneurysm | Standard | AD | Unassigned |
|
Fibrillin-1 | Yes | + | + | Marfan syndrome | ARD, TAAD, AAA, other arterial aneurysms, pulmonary artery dilatation, arterial tortuosity | 5.0 | AD | 154700 |
|
Fibrillin-2 | No | + | − | Contractural arachnodactyly | Rare ARD and aortic dissection, BAV, PDA | Standard | AD | 121050 |
|
Filamin A | Yes | + | − | Periventricular nodular heterotopia | Aortic dilatation/aneurysms, peripheral arterial dilatation, PDA, IA, BAV | Standard | XLD | 300049 |
|
Forkhead box 3 | Yes | − | + | AAT11 | TAAD (primarily type A dissection) | Standard | AD | 617349 |
|
Lysyl oxidase | Yes | − | + | AAT10 | TAAD, AAA, hepatic artery aneurysm, BAV, CAD | Standard | AD | 617168 |
|
Methionine adenosyltransferase II alpha | No | − | + | FTAA | Thoracic aortic aneurysms, BAV | Standard | AD | Unassigned |
|
Microfibril-associated glycoprotein 2 | Partially | − | + | AAT9 | ARD, TAAD | Standard | AD | 616166 |
|
Smooth muscle myosin heavy chain | Partially | − | + | AAT4 | TAAD, early aortic dissection, PDA, CAD, peripheral vascular occlusive disease, carotid IA | 4.5–5.0 | AD | 132900 |
|
Myosin light chain kinase | No | − | + | AAT7 | TAAD, early aortic dissections | 4.5–5.0 | AD | 613780 |
|
NOTCH1 | Partially | − | + | AOVD1 | BAV/TAAD | Standard | AD | 109730 |
|
Type 1 cGMP-dependent protein kinase | No | − | + | AAT8 | TAAD, early aortic dissection, AAA, coronary artery aneurysm/dissection, aortic tortuosity, small vessel CVD | 4.5–5.0 | AD | 615436 |
|
Sloan Kettering proto-oncoprotein | No | + | − | Shprintzen-Goldberg syndrome | ARD, arterial tortuosity, pulmonary artery dilation, other (splenic) arterial aneurysms | Standard | AD | 182212 |
|
Glucose transporter 10 | No | + | − | Arterial tortuosity syndrome | ARD, ascending aortic aneurysms, other arterial aneurysms, arterial tortuosity, elongated arteries aortic/pulmonary artery stenosis | Standard | AR | 208050 |
|
SMAD2 | No | + | − | Unidentified CTD with arterial aneurysm/dissections | ARD, ascending aortic aneurysms, vertebral/carotid aneurysms and dissections, AAA | Standard | AD | Unassigned |
|
SMAD3 | Partially | + | + | LDS type 3 | ARD, TAAD, early aortic dissection, AAA, arterial tortuosity, other arterial aneurysms/dissections, IA, BAV | 4.0–4.2 | AD | 613795 |
|
SMAD4 | Yes | + | − | JP/HHT syndrome | ARD, TAAD, AVMs, IA | Standard | AD | 175050 |
|
SMAD6 | No | − | + | AOV2 | BAV/TAA | Standard | AD | 602931 |
|
TGF-β2 | Yes | + | + | LDS type 4 | ARD, TAAD, arterial tortuosity, other arterial aneurysms, BAV | 4.5–5.0 | AD | 614816 |
|
TGF-β3 | No | + | − | LDS type 5 | ARD, TAAD, AAA/dissection, other arterial aneurysms, IA/dissection | Standard | AD | 615582 |
|
TGF-β receptor type 1 | Yes | + | + | LDS type 1 + AAT5 | TAAD, early aortic dissection, AAA, arterial tortuosity, other arterial aneurysms/dissection, IA, PDA, BAV | 4.0–4.5 | AD | 609192 |
|
TGF-β receptor type 2 | Yes | + | + | LDS type 2 + AAT3 | TAAD, early aortic dissection, AAA, arterial tortuosity, other arterial aneurysms/dissection, IA, PDA, BAV | 4.0–4.5 | AD | 610168 |
EDS, Ehlers-Danlos syndrome; LDS, Loyez-Dietz syndrome; TAAD, thoracic aortic aneurysm and dissection; AD, autosomal dominant; AR, autosomal recessive; CTD, connective tissue disease; JP, juvenile polyposis; HHT, hereditary hemorrhagic telangiectasia; PDA, patent ductus arteriosus; CAD, coronary artery disease; BAV, bicuspid aortic valve; ARD, aortic root dilatation; SVAS, supravalvular aortic stenosis; AAA, abdominal aortic aneurysm; OMIM, Online Mendelian Inheritance in Man.
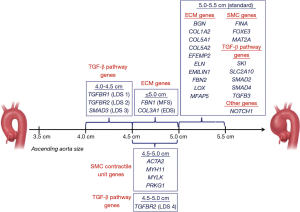
TGF-β signaling: TGFBR1 and TGFBR2, TGF-β2 and SMAD3
In addition to their involvement in LDS pathogenicity, TGFBR1 and TGFBR2 also contribute to the development of the non-syndromic form of TAAD. Specifically, TGFBR1 mutations are found in the familial thoracic aortic aneurysm-5 (AAT5) and TGFBR2 mutation—in the familial thoracic aortic aneurysm-3 (AAT3). The molecular mechanism in both cases involve dysregulation of the TGF-β signaling pathway due to impaired receptor function. For patients with mutation in TGFBR2, surgery is recommended when the diameter reaches 4.2 cm. This recommendation applies to both for LDS and FTAAD patients with this mutation (2,19). Additionally, patients carrying mutations in the TGFBR2 gene are more likely to develop aortic dissection in the absence of significant aortic enlargement as compared to the patients with the TGFBR1 gene mutations (20).
Similar to TGFBR1 and 2, TGF-β2 and SMAD3, in addition to producing non-syndromic TAA, may also be present in syndromic TAA as part of LDS. They are also involved in the TGF-β signaling where the TGF-β2 gene encodes the ligand for the TGFB receptor and SMAD3 gene produces the downstream phosphorylation target for the TGFBR. Patients with these mutations are at high risk of dissection when the aortic diameter is less than 5.0 cm. Therefore, surgical intervention is recommended if the diameter is greater than 4.5 cm, especially if patients have a familial history of aortic dissection, rapid growth, or have been scheduled for aortic valve replacement surgery (20).
Smooth muscle contractile unit: MYH11, ACTA2, MYLK and PRKG1
This group of genes contribute to FTAAD pathogenesis by affecting structure or function of smooth muscle cells. The product of the MYH11 gene is myosin heavy chain protein, which, together with another smooth muscle protein, actin, produces mechanical force for muscle contraction. Mutations in the MYH11 gene tend to generate a dominant negative version of the protein and demonstrate an incomplete penetrance. Moreover, the presence of the MYH11 mutation does not always correlate with the presence of the disease, which suggests that there could be other effectors contributing to the phenotype development, possibly in a synergistic manner (21). Current recommendation for intervention is to undergo surgery when the aortic diameter is between 4.5 and 5.0 cm (21,22).
The ACTA2 gene encodes alpha smooth muscle actin protein, a transcriptional target of the TGF-β signaling pathway, which is also involved in smooth muscle contraction. To become functional, actin filaments must polymerize with each other via specific amino acid residues. Mutations within the ACTA2 locus that change those amino acids tend to produce a dominant negative protein that is unable to polymerize (23). Since patients with mutations in ACTA2 tend to have aortic dissection at diameter less than 5.0 cm, surgical intervention should be considered when the aortic diameter is between 4.5 and 5.0 cm (23,24).
The product of the MYLK gene is myosin light chain kinase, which activates myosin via phosphorylation, allowing for smooth muscle contraction. The disease-causing mutation in this gene produces a fully non-functional protein as it tends to destroy the binding site for calmodulin, a partner necessary for kinase phosphorylation activity (25). Similar to other smooth muscle contractile unit genes, patients with MYLK mutations have dissections at an aortic diameter less than 5.0 cm and should undergo surgical intervention when the size is between 4.5 and 5.0 cm (21). However, the fact that mutation in the MYLK gene can at times present with ascending aortic dissection with minimal enlargement of the aortic diameter should also be taken into consideration when making recommendations for surgical repair (26).
Another gene contributing to non-syndromic TAAD via altering smooth muscle cell contraction is PRKG1. This gene encodes the type 1 cGMP-dependent protein kinase that is involved in the process of the smooth muscle relaxation and works by altering smooth muscle contraction (26). Heterozygous mutations in this gene locus are associated with familial thoracic aortic aneurysm-8 (AAT8) and contribute to the loss and fragmentation of elastic fibers, decreased amount of smooth muscle cells, and invasion of the vasa vasorum into the medial layer (26). Surgical repair for patients with the mutations in this gene is normally recommended when the ascending aortic size is 4.5–5.0 cm.
Conclusions
Genetic mutations involving TGF-β signaling pathway, smooth muscle cell function and the structural integrity of the extracellular matrix contribute to TAAD development and progression. The connection between the TAAD heterogeneous molecular genetic profile and the different levels of TAAD severity strongly supports the use of gene sequencing in patient care. We and others have expended on and reported large clinical experience with exome sequencing in thoracic aortic care. Genetic testing should indeed modulate when to operate in thoracic aortic disease. Such testing permits focused, precise, truly personalized thoracic aortic care.
Acknowledgments
Funding: None.
Footnote
Provenance and Peer Review: This article was commissioned by the Guest Editor (Mohamad Bashir) for the series “The 2nd Barts Aortovascular Symposium” published in Journal of Visualized Surgery. The article has undergone external peer review.
Conflicts of Interest: All authors have completed the ICMJE uniform disclosure form (available at http://dx.doi.org/10.21037/jovs.2018.07.14). The series “The 2nd Barts Aortovascular Symposium” was commissioned by the editorial office without any funding or sponsorship. The authors have no other conflicts of interest to declare.
Ethical Statement: The authors are accountable for all aspects of the work in ensuring that questions related to the accuracy or integrity of any part of the work are appropriately investigated and resolved.
Open Access Statement: This is an Open Access article distributed in accordance with the Creative Commons Attribution-NonCommercial-NoDerivs 4.0 International License (CC BY-NC-ND 4.0), which permits the non-commercial replication and distribution of the article with the strict proviso that no changes or edits are made and the original work is properly cited (including links to both the formal publication through the relevant DOI and the license). See: https://creativecommons.org/licenses/by-nc-nd/4.0/.
References
- Danyi P, Elefteriades JA, Jovin IS. Medical therapy of thoracic aortic aneurysms: are we there yet? Circulation 2011;124:1469-76. [Crossref] [PubMed]
- Hiratzka LF, Bakris GL, Beckman JA, et al. 2010 ACCF/AHA/AATS/ACR/ASA/SCA/SCAI/SIR/STS/SVM guidelines for the diagnosis and management of patients with Thoracic Aortic Disease: a report of the American College of Cardiology Foundation/American Heart Association Task Force on Practice Guidelines, American Association for Thoracic Surgery, American College of Radiology, American Stroke Association, Society of Cardiovascular Anesthesiologists, Society for Cardiovascular Angiography and Interventions, Society of Interventional Radiology, Society of Thoracic Surgeons, and Society for Vascular Medicine. Circulation 2010;121:e266-369. [Crossref] [PubMed]
- Albornoz G, Coady MA, Roberts M, et al. Familial thoracic aortic aneurysms and dissections--incidence, modes of inheritance, and phenotypic patterns. Ann Thorac Surg 2006;82:1400-5. [Crossref] [PubMed]
- Pearson GD, Devereux R, Loeys B, et al. Report of the National Heart, Lung, and Blood Institute and National Marfan Foundation Working Group on research in Marfan syndrome and related disorders. Circulation 2008;118:785-91. [Crossref] [PubMed]
- Bradley TJ, Alvarez NA, Horne SG. A Practical Guide to Clinical Management of Thoracic Aortic Disease. Can J Cardiol 2016;32:124-30. [Crossref] [PubMed]
- Loeys BL, Schwarze U, Holm T, et al. Aneurysm syndromes caused by mutations in the TGF-beta receptor. N Engl J Med 2006;355:788-98. [Crossref] [PubMed]
- Meester JAN, Verstraeten A, Schepers D, et al. Differences in manifestations of Marfan syndrome, Ehlers-Danlos syndrome, and Loeys-Dietz syndrome. Ann Cardiothorac Surg 2017;6:582-94. [Crossref] [PubMed]
- Jondeau G, Ropers J, Regalado E, et al. International Registry of Patients Carrying TGFBR1 or TGFBR2 Mutations: Results of the MAC (Montalcino Aortic Consortium). Circ Cardiovasc Genet 2016;9:548-58. [Crossref] [PubMed]
- Renard M, Callewaert B, Malfait F, et al. Thoracic aortic-aneurysm and dissection in association with significant mitral valve disease caused by mutations in TGFB2. Int J Cardiol 2013;165:584-7. [Crossref] [PubMed]
- MacCarrick G, Black JH 3rd, Bowdin S, et al. Loeys-Dietz syndrome: a primer for diagnosis and management. Genet Med 2014;16:576-87. [Crossref] [PubMed]
- Andelfinger G, Loeys B, Dietz H. A Decade of Discovery in the Genetic Understanding of Thoracic Aortic Disease. Can J Cardiol 2016;32:13-25. [Crossref] [PubMed]
- Brownstein AJ, Ziganshin BA, Kuivaniemi H, et al. Genes Associated with Thoracic Aortic Aneurysm and Dissection: An Update and Clinical Implications. Aorta (Stamford) 2017;5:11-20. [Crossref] [PubMed]
- Byers PH, Belmont J, Black J, et al. Diagnosis, natural history, and management in vascular Ehlers-Danlos syndrome. Am J Med Genet C Semin Med Genet 2017;175:40-47. [Crossref] [PubMed]
- Coucke PJ, Willaert A, Wessels MW, et al. Mutations in the facilitative glucose transporter GLUT10 alter angiogenesis and cause arterial tortuosity syndrome. Nat Genet 2006;38:452-7. [Crossref] [PubMed]
- Santoro G, Caianiello G, Rossi G, et al. Hybrid transcatheter-surgical strategy in arterial tortuosity syndrome. Ann Thorac Surg 2008;86:1682-4. [Crossref] [PubMed]
- Syu YW, Lai HW, Jiang CL, et al. GLUT10 maintains the integrity of major arteries through regulation of redox homeostasis and mitochondrial function. Hum Mol Genet 2018;27:307-21. [Crossref] [PubMed]
- Siddiqui S, Rana Y, Patel H, et al. Ascending Aortic Aneurysm in a Case of Arterial Tortuosity Syndrome: A Rare Tortuous Disorder. World J Pediatr Congenit Heart Surg 2017; [Epub ahead of print]. [Crossref] [PubMed]
- Ritelli M, Chiarelli N, Dordoni C, et al. Arterial Tortuosity Syndrome: homozygosity for two novel and one recurrent SLC2A10 missense mutations in three families with severe cardiopulmonary complications in infancy and a literature review. BMC Med Genet 2014;15:122. [Crossref] [PubMed]
- Tran-Fadulu V, Pannu H, Kim DH, et al. Analysis of multigenerational families with thoracic aortic aneurysms and dissections due to TGFBR1 or TGFBR2 mutations. J Med Genet 2009;46:607-13. [Crossref] [PubMed]
- Campens L, Renard M, Callewaert B, et al. New insights into the molecular diagnosis and management of heritable thoracic aortic aneurysms and dissections. Pol Arch Med Wewn 2013;123:693-700. [PubMed]
- Harakalova M, van der Smagt J, de Kovel CG, et al. Incomplete segregation of MYH11 variants with thoracic aortic aneurysms and dissections and patent ductus arteriosus. Eur J Hum Genet 2013;21:487-93. [Crossref] [PubMed]
- Gillis E, Van Laer L, Loeys BL. Genetics of thoracic aortic aneurysm: at the crossroad of transforming growth factor-β signaling and vascular smooth muscle cell contractility. Circ Res 2013;113:327-40. [Crossref] [PubMed]
- Isselbacher EM, Lino Cardenas CL, Lindsay ME. Hereditary Influence in Thoracic Aortic Aneurysm and Dissection. Circulation 2016;133:2516-28. [Crossref] [PubMed]
- Regalado ES, Guo DC, Prakash S, et al. Aortic Disease Presentation and Outcome Associated With ACTA2 Mutations. Circ Cardiovasc Genet 2015;8:457-64. [Crossref] [PubMed]
- Wang L, Guo DC, Cao J, et al. Mutations in myosin light chain kinase cause familial aortic dissections. Am J Hum Genet 2010;87:701-7. [Crossref] [PubMed]
- Guo DC, Regalado E, Casteel DE, et al. Recurrent gain-of-function mutation in PRKG1 causes thoracic aortic aneurysms and acute aortic dissections. Am J Hum Genet 2013;93:398-404. [Crossref] [PubMed]
Cite this article as: Kostiuk V, Brownstein AJ, Ziganshin BA, Elefteriades JA. Genetic testing to modulate when to operate in thoracic aortic disease. J Vis Surg 2018;4:193.