Preoperative planning of thoracic surgery with use of three-dimensional reconstruction, rapid prototyping, simulation and virtual navigation
Introduction
For the past two decades, the complexity of interventions in surgery in general and thoracic surgery specifically has increased. Due to the development of more precise diagnostic possibilities, thoracic disease is being diagnosed in an earlier phase. Especially within computed tomography (CT) technology, several innovations, such as the helical CT-scan, multi-detector CT-scan and high-resolution CT-scan, have led to the early-phase detection (1). Together with the rise of minimally invasive surgery as a standard treatment for early-phase disease, procedures tend to require more skills and precision (2).
At the same time, the European Working Time Directive (EWTD) limits the working hours of surgeons and residents (3). In an era where Operating Room (OR)-time is more valuable and ethical considerations play a more important role, residents and surgeons have less possibilities to develop their surgical skills intra-operatively (4,5). Therefore, precise preoperative planning and simulation based learning are indispensable.
On the other hand, we are experiencing a paradigm shift from uni-disciplinary to multidisciplinary medicine with a central role for the patient. The focus will shift from whether a certain treatment is better than other to which patient will benefit the most from which technique?
This article will focus on this paradigm shift and will graphically present the new diagnostic and therapeutic possibilities in thoracic surgery facilitating this concept.
New diagnostical techniques
Fiberoptic bronchoscopy has been the standard diagnostic approach for detection of pulmonary lesions (6). However, the bronchoscope with a 5–6 mm diameter is limited to the third and fourth-order bronchi. With the introduction of the ultra-thin bronchoscope, peripheral pulmonary lesions can more easily be detected. Nonetheless, navigation through the complex bronchial tree on direct vision remains difficult and mistakes are easily being made. Virtual bronchoscopy (VB) however, allows the physician to have a three-dimensional (3D) understanding of the bronchial tree based on thin-slice helical CT images (7). Figure 1 demonstrates the VB-guided ultra-thin bronchoscope in the detection of peripheral pulmonary lesions. This approach allows the physician to precisely detect early-phase peripheral disease. A valuable update of this technique was the addition of special software that corrected for rotation of the bronchoscope on the VB image.
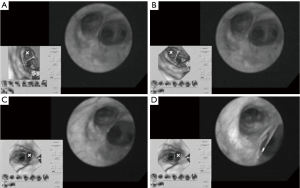
Recently, the introduction of electromagnetic (EM) navigation to VB has even enhanced this technique and allows the physician to have an optimal understanding of the bronchoscopes position. EM navigated VB uses the same CT-imaging techniques, however the patient is situated in an EM-field and there is a location sensor placed on the tip of the flexible bronchoscope. The exact location of the tip is depicted on the CT-images, which are superimposed on the real anatomy of the patient due to matching of actual and virtual bronchoscopic views (8).
With the emergence of other imaging modalities, of which the endobronchial ultrasound (EBUS) is most important, peripheral pulmonary lesions can more easily be assessed and staged after CT detection of a potential pulmonary lesion (9). The radial EBUS probe is advanced through a guide-sheath into the bronchi. In the more proximal bronchi, a water-filled balloon is inflated around the probe to optimize image quality. The probe will make direct contact with the bronchus wall in more peripheral bronchi. With use of EBUS, bronchial wall infiltration can be assessed. After visual assessment, the EBUS is removed from the guide-sheath and a needle for transbronchial biopsy (TBB) or transbronchial needle aspiration (TBNA) is advanced through the guide-sheath. Specimens for pathology examination can be obtained precisely by use of this technique. In certain mediastinal lesions, specimens can be collected by utility of esophageal ultrasound (EUS)-guided TBB or TBNA. The diagnostic process and evaluation of the peripheral pulmonary lesion with utility of EBUS is depicted in Figure 2.
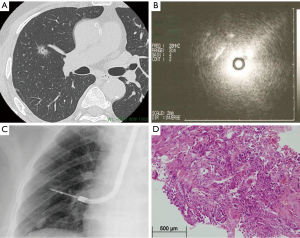
All together, these new imaging modalities give us the possibility to detect thoracic disease in an earlier phase and in a more peripheral region of the lung. However, smaller operating areas, together with the emergence of minimally invasive techniques such as video-assisted thoracoscopic surgery (VATS), require more skilled surgeons, comprehensive preoperative planning and extensive training for surgical residents. Subsequently, we are experiencing a shift from conventional surgery to image-guided surgery for thoracic disease.
Image-guided surgery
3D-reconstruction
Different techniques for image-guided thoracic surgery exist. In our center (Maastricht University Medical Center, Maastricht, the Netherlands) we use 3D CT reconstruction software (Vesalius 3D, PS-Medtech, Amsterdam, the Netherlands). This technique has been described elsewhere (10). With utility of this software program we are able to segment different tissues from each other. Through this method we are able to assess the exact location of the lesion in the thorax (Figure 3). Furthermore, we are able to determine the exact lung segment with its affiliated vessel and bronchial branches and determine the ideal incision level for VATS segmentectomy or lobectomy (Figure 4). Finally, we are able to segment these branches from each other and assess the designated pulmonary artery, vein and bronchus, enabling us to detect potential vascular or bronchial anomalies (Figure 5).
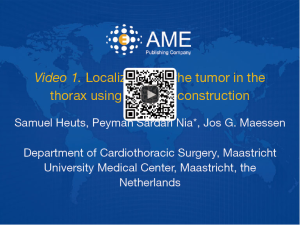
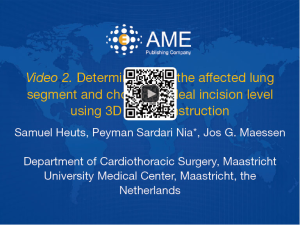
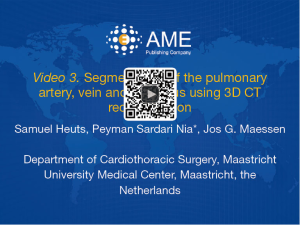
Ikeda et al. presented a similar preoperative planning technique with use of 3D CT reconstruction models in which they confirm port placement for VATS and identify the designated pulmonary artery and vein (Figure 6). They found an overall detection rate of 95–97% of the pulmonary arteries in preoperative planning of VATS lobectomy or segmentectomy (14).
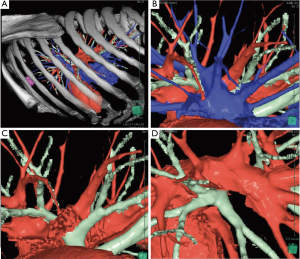
Marking of pulmonary lesions
Especially for small pulmonary nodules, it can be difficult to detect them during thoracoscopic procedures. Therefore, preoperative localization and marking of these lesions can be useful to create a sort of pathway for the surgeon to the lesion and to be able to resect within the correct surgical margins without resecting to much pulmonary tissue.
Toba et al. described pulmonary lesion marking with use of a metallic coil through VB-guided bronchoscopy. They studied 154 patients with 161 lesions (15). Thin-slice CT identified the pulmonary lesion after which VB was created. VB-guided bronchoscopy led the physician to the designated bronchus after which the metallic coil was placed under fluoroscopy guidance with a tracheobronchial coil-feeding catheter over the tip of the bronchoscope. After placement of the coil, the relationship between the pulmonary lesion and the coil was checked by CT again. The investigators did not report the mean time between placement of the coil and surgery, however, they assumed an intra-bronchial placed coil would not migrate. Trocars were inserted under fluoroscopy guidance and VATS was performed. Finally, the nodule with the metallic coil could be resected and the specimen was examined by the pathologist immediately in order to determine a margin-free resection. This whole process is described and depicted in Figure 7.
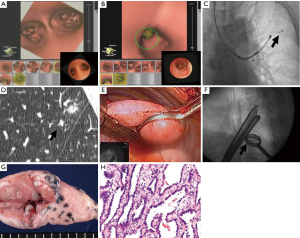
Similar techniques have been used in other centers. Suzuki et al. used hook wires instead of metallic coils and they reached a technical success rate of 97.5% (16). Likewise, Gobardhan et al. placed intra-lesional iodine-125 seeds under CT-guidance with a success rate of 78.6% (17).
Another variance reported by Gill et al. was with so-called image-guided video-assisted thoracoscopic surgery (iVATS) in a prospective clinical trial with 23 patients (18). Pulmonary nodule identification was again established with preoperative CT-scans. A 3D reconstruction was made to assess the relationship of the tumor with the surrounding osseous structures. However, in this study, patients were brought into a special advanced multimodality image guided operating (AMIGO) room. General anesthesia was administered and a C-arm CT-scan was performed to precisely identify the nodule, after which the radiologist planned a percutaneous pathway for T-bar placement in the nodule under fluoroscopy with needle guidance software using laser and guidance markers. Subsequently, the patients underwent iVATS wedge resection. The wedge resection was then first evaluated with a CT-scan and afterwards a frozen section histological analysis was performed. This process is elaborated in Figure 8.
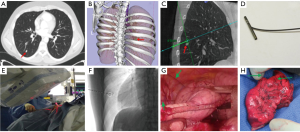
Staining of pulmonary lesions
Another method of preoperative physical characterization of the lesion is by staining. Sato et al. named this method virtual assisted lung mapping (VAL-MAP). They studied 100 consecutive patients with 156 lesions in a single institute (19). The VAL-MAP procedure was designed to identify non-palpable lesions and to determine ideal wedge resection lines. A preoperative CT-scan identified the lesion after which a 3D reconstruction was made. As in the study by Toba et al. VB-guided bronchoscopy was used to reach the designated bronchus. However, in this study, they did not place a metallic coil, but the lesion was transbronchially stained under fluoroscopy guidance with 1 mL of indigo-carmine. Staining was first evaluated by a post-VAL-MAP CT-scan and subsequently VATS resection was performed. Figure 9 depicts the process from pre-VAL-MAP CT-scan to intra-operative VATS-view.
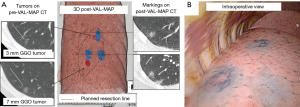
A related technique was described by Shentu et al. in their study of 74 patients with 80 peripheral pulmonary lesions (20). In this study, patients first underwent a virtual puncture for staining with utility of a radiotherapy planning simulator on the day before the operation. On the day of the operation methylene blue was injected percutaneously according to the virtual puncture, with a localization success rate of 94%, after which VATS was performed.
Virtual reality
Several types of virtual reality aided techniques exist. Firstly, surgery can be performed preoperatively on a virtual simulator, as Saji et al. presented. They reported the use of virtual segmentectomy, surgical simulation of a segmentectomy using 3D modelling (21). A 3D reconstruction was established from multi-detector CT Angiography images and was loaded into special simulation software. The 3D reconstruction was assessed by the surgeon and corrected if necessary for anatomical variability. The tumor was virtually reconstructed together with the pulmonary vessels and bronchi after which the designated lung segment was determined. The bronchial ventilation area was then calculated and the resection lines for vessels, bronchi and inter-segmental veins were chosen. Afterwards, the extent of the surgical margin was calculated as presented in Figure 10. Finally the actual 3D CT-guided real-time segmentectomy was performed using VATS. None of the patients had recurrence of the tumor after a follow-up of 12–14 months.
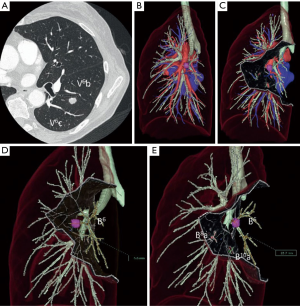
Another application of virtual reality is with use of intra-operative virtual reality stereo-navigation. Kanzaki et al. presented their experience with binocular stereo-navigation during VATS in ten patients (22). All patients underwent a high-resolution CT-scan of the tumor and hilum, which were converted to a 3D reconstruction and loaded into a personal computer on which virtual reality software was installed. All important pulmonary structures (i.e., arteries, veins, tumor and bronchi) were marked by the surgeon and an anatomical correct model was reconstructed (Figure 11). This anatomical model was then uploaded into special software which was installed on hardware connected to a 3D screen. The 3D screen was intra-operatively set up next to the monitor for VATS surgery (Figure 12). Surgeons were wearing special 3D glasses which allowed them to access the 3D screen at any given moment during surgery. Postoperatively, none of the patients had macroscopically positive surgical margins.
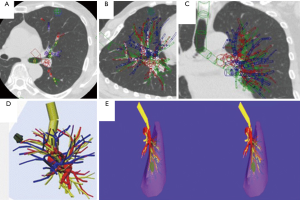
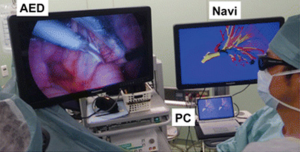
Rapid prototyping (RP)
The most recent technique used for preoperative planning of thoracic surgery is RP, also known as 3D printing. For the past years application of this technique has been growing exponentially. In maxillo-facial and orthopedic surgery, this technique has been used for a while now. These medical disciplines already use the rigid RP’s for therapeutic reasons. However, in the thoracic specialty and organ-related disciplines, this technique is still in its infancy. In these disciplines, few reports of RP are used for diagnostic and simulation reasons. Akiba et al. presented their experience with RP in preoperative planning of the fissure less technique for VATS segmentectomy/lobectomy in two cases (23). A 3D printer was used to produce the mixed color RP’s. Information was derived from contrast-enhanced CT-scans which underwent volume analysis in special-designed software. Bronchi and vessels were being colored in different ways, allowing the surgeon distinguish them from each other. Therefore, anatomical deviations in the pulmonary branches could more easily be detected. Figure 13 shows the RP and compares this to the intra-operative view.
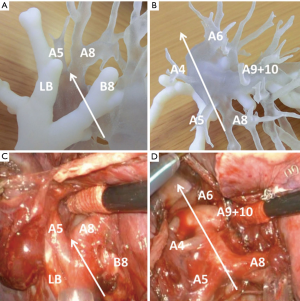
Conclusions
Several innovations in diagnostic tools have led to more early-phase disease detection. Thereby, new, less invasive techniques have emerged for its treatment. However, these new techniques require more skill of surgeons. Together with the new concept of patient-central personalized medicine, preoperative planning of these operations is crucial and indispensable.
Several different techniques within preoperative planning of thoracic surgery facilitate the shift to this concept. We believe that preoperative planning and simulation will increase the effectivity and reproducibility of surgical interventions and subsequently increasing patient safety (5).
Indeed, several studies have shown that surgical simulation significantly improves residents’ skill level and lead to less intraoperative errors (24-26). Additionally, surgical effectivity will increase, illustrated by shorter operating times, better surgical results, less complication and less reoperation. Finally, the goal of preoperative planning and simulation is to make operations reproducible. By increasing surgical reproducibility, we will be able to compare techniques, standardize procedures and establish procedural superiority.
Preoperative planning and simulation already are a very important part of surgery in many centers and we believe this will only increase. In our opinion, virtual reality simulation will have a special and valuable place within this concept and we consider this to be the most important and effective preoperative planning method for the future.
Acknowledgements
None.
Footnote
Conflicts of Interest: The authors have no conflicts of interest to declare.
References
- Henschke CI, McCauley DI, Yankelevitz DF, et al. Early lung cancer action project: a summary of the findings on baseline screening. Oncologist 2001;6:147-52. [Crossref] [PubMed]
- Petersen RH, Hansen HJ. Learning curve associated with VATS lobectomy. Ann Cardiothorac Surg 2012;1:47-50. [PubMed]
- Schimmack S, Hinz U, Wagner A, et al. Maximizing time from the constraining European Working Time Directive (EWTD): The Heidelberg New Working Time Model. Health Econ Rev 2014;4:14. [Crossref] [PubMed]
- Niederee MJ, Knudtson JL, Byrnes MC, et al. A survey of residents and faculty regarding work hour limitations in surgical training programs. Arch Surg 2003;138:663-9; discussion 669-71. [Crossref] [PubMed]
- Reznick RK, MacRae H. Teaching surgical skills--changes in the wind. N Engl J Med 2006;355:2664-9. [Crossref] [PubMed]
- Torrington KG, Kern JD. The utility of fiberoptic bronchoscopy in the evaluation of the solitary pulmonary nodule. Chest 1993;104:1021-4. [Crossref] [PubMed]
- Asano F, Matsuno Y, Shinagawa N, et al. A virtual bronchoscopic navigation system for pulmonary peripheral lesions. Chest 2006;130:559-66. [Crossref] [PubMed]
- Gex G, Pralong JA, Combescure C, et al. Diagnostic yield and safety of electromagnetic navigation bronchoscopy for lung nodules: a systematic review and meta-analysis. Respiration 2014;87:165-76. [Crossref] [PubMed]
- Chavez C, Sasada S, Izumo T, et al. Endobronchial ultrasound with a guide sheath for small malignant pulmonary nodules: a retrospective comparison between central and peripheral locations. J Thorac Dis 2015;7:596-602. [PubMed]
- Heuts S, Maessen JG, Sardari Nia P. Preoperative planning of left-sided valve surgery with 3D computed tomography reconstruction models: sternotomy or a minimally invasive approach?†. Interact Cardiovasc Thorac Surg 2016. [Epub ahead of print]. [Crossref] [PubMed]
- Heuts S, Sardari Nia P, Maessen JG. Localization of the tumor in the thorax using 3D CT reconstruction. Asvide 2016;3:113. Available online: http://www.asvide.com/articles/867
- Heuts S, Sardari Nia P, Maessen JG. Determination of the affected lungsegment and choice for ideal incision level using 3D CT reconstruction. Asvide 2016;3:114. Available online: http://www.asvide.com/articles/868
- Heuts S, Sardari Nia P, Maessen JG. Segmentation of the pulmonary artery, vein and bronchus using 3D CT reconstruction. Asvide 2016;3:115. Available online: http://www.asvide.com/articles/869
- Ikeda N, Yoshimura A, Hagiwara M, et al. Three dimensional computed tomography lung modeling is useful in simulation and navigation of lung cancer surgery. Ann Thorac Cardiovasc Surg 2013;19:1-5. [Crossref] [PubMed]
- Toba H, Kondo K, Miyoshi T, et al. Fluoroscopy-assisted thoracoscopic resection after computed tomography-guided bronchoscopic metallic coil marking for small peripheral pulmonary lesions. Eur J Cardiothorac Surg 2013;44:e126-32. [Crossref] [PubMed]
- Suzuki K, Shimohira M, Hashizume T, et al. Usefulness of CT-guided hookwire marking before video-assisted thoracoscopic surgery for small pulmonary lesions. J Med Imaging Radiat Oncol 2014;58:657-62. [Crossref] [PubMed]
- Gobardhan PD, Djamin RS, Romme PJ, et al. The use of iodine seed (I-125) as a marker for the localisation of lung nodules in minimal invasive pulmonary surgery. Eur J Surg Oncol 2013;39:945-50. [Crossref] [PubMed]
- Gill RR, Zheng Y, Barlow JS, et al. Image-guided video assisted thoracoscopic surgery (iVATS) - phase I-II clinical trial. J Surg Oncol 2015;112:18-25. [Crossref] [PubMed]
- Sato M, Yamada T, Menju T, et al. Virtual-assisted lung mapping: outcome of 100 consecutive cases in a single institute. Eur J Cardiothorac Surg 2015;47:e131-9. [Crossref] [PubMed]
- Shentu Y, Zhang L, Gu H, et al. A new technique combining virtual simulation and methylene blue staining for the localization of small peripheral pulmonary lesions. BMC Cancer 2014;14:79. [Crossref] [PubMed]
- Saji H, Inoue T, Kato Y, et al. Virtual segmentectomy based on high-quality three-dimensional lung modelling from computed tomography images. Interact Cardiovasc Thorac Surg 2013;17:227-32. [Crossref] [PubMed]
- Kanzaki M, Isaka T, Kikkawa T, et al. Binocular stereo-navigation for three-dimensional thoracoscopic lung resection. BMC Surg 2015;15:56. [Crossref] [PubMed]
- Akiba T, Nakada T, Inagaki T. Simulation of the fissureless technique for thoracoscopic segmentectomy using rapid prototyping. Ann Thorac Cardiovasc Surg 2015;21:84-6. [Crossref] [PubMed]
- Seymour NE, Gallagher AG, Roman SA, et al. Virtual reality training improves operating room performance: results of a randomized, double-blinded study. Ann Surg 2002;236:458-63; discussion 463-4. [Crossref] [PubMed]
- Van Sickle KR, Ritter EM, Baghai M, et al. Prospective, randomized, double-blind trial of curriculum-based training for intracorporeal suturing and knot tying. J Am Coll Surg 2008;207:560-8. [Crossref] [PubMed]
- Ahlberg G, Enochsson L, Gallagher AG, et al. Proficiency-based virtual reality training significantly reduces the error rate for residents during their first 10 laparoscopic cholecystectomies. Am J Surg 2007;193:797-804. [Crossref] [PubMed]
Cite this article as: Heuts S, Sardari Nia P, Maessen JG. Preoperative planning of thoracic surgery with use of three-dimensional reconstruction, rapid prototyping, simulation and virtual navigation. J Vis Surg 2016;2:77.